Research
Our lab is interested in understanding the mechanisms that govern cell fate decisions, particularly in epithelial stem cell lineages. In many epithelial tissues, stem cells divide continuously to self-renew and produce daughter cells that differentiate into functional cell types of the tissue. The production of new cells and the specification of stem cell and daughter cell identity is a remarkable process that must be flexible enough to accommodate changing tissue demands, and yet robust enough to ensure that each cell type is specified in the correct time and place. This process occurs spontaneously, and important cell fate decisions are made within discrete regions of the tissue but add up to a cohesive whole, much like ants distributing tasks at the individual level to meet the needs of the entire colony. We use the Drosophila ovary, the mouse intestinal epithelium and other experimental models to understand how this process works.
Stem cell niche competition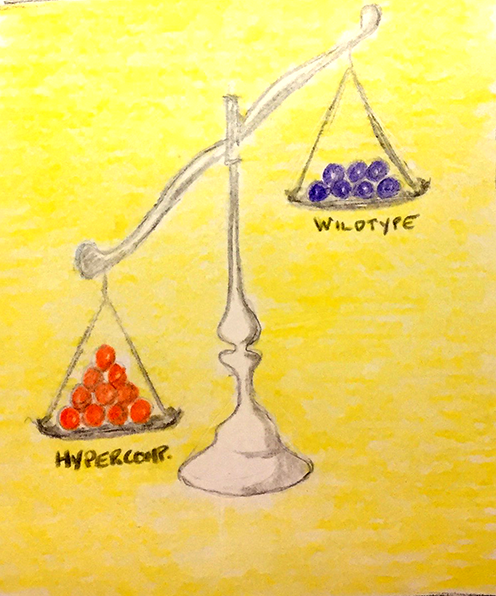
Epithelial stem cells are not immortal. Indeed, during normal adult homeostasis, epithelial stem cells are regularly lost from the tissue and replaced by other nearby cells. The cause of stem cell loss and the basis for selection of a replacement cell is not fully understood, but it is now clear that one important mechanism involves competition for the stem cell niche. [1], [2]
We found that the pattern of follicle stem cell loss and replacement in a wildtype Drosophila ovary can be described by a neutral competition model, and we have identified several mutations that cause non-neutral or “biased” competition, in which the mutant and wildtype stem cells are replaced at unequal rates. Some mutations cause the mutant stem cell to become more competitive for the niche than wildtype (hypercompetition) whereas other mutations cause the mutant stem cell to become less competitive than wildtype (hypocompetition). We are now using these mutants to understand the genetic basis of niche competition. Through collaborations, we are expanding the mathematical framework of the the stem cell niche competition model and extending our studies to the mouse intestinal epithelium.
The epithelial stem cell niche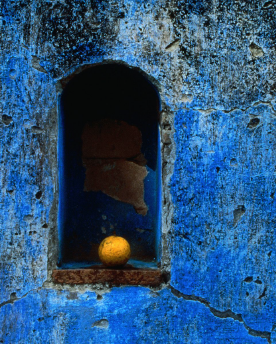
Adult stem cells reside in distinct microenvironments, or niches, that promote self-renewal and regulate stem cell behavior. Niches have been hard to study in vivo because it is often difficult to precisely identify and genetically manipulate the stem cells and niche cells. To understand the follicle stem cell niche, we first developed new genetic tools [1] and identified the source of key niche signals [2].
Surprisingly, we have found that the follicle stem cell niche does not have a fixed position within the tissue and at least some cellular components of the niche turn over regularly during adult life. In addition, we found that Wnt signaling, which is essential for follicle stem cell self-renewal, is active only intermittently, suggesting that niche signaling is not static. We are using live imaging, new signaling pathway reporters, and quantitative 3D fluorescence microscopy to understand how this niche is able to function with precision amid a dynamic tissue environment.
Transit amplifying cells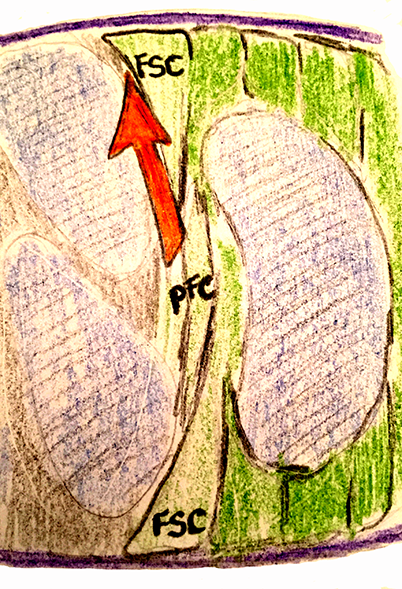
In many epithelial tissues, newly produced daughter cells differentiate slowly, over the course of several divisions [1]. This “transit amplifying” stage is poorly understood. Although transit amplifying cells have exited the niche and thus are no longer receiving niche signals, at least some retain the capacity to re-enter the niche and acquire the stem cell fate.
However, many cells in the transit amplifying population are receiving new signals that direct them down a path toward differentiation. This suggests that there is heterogeneity in the transit amplifying population, but little is known about whether or how transit amplifying cells differ from one another. We discovered a mechanism that allows newly produced transit amplifying cells in the follicle stem cell lineage to retain a molecular memory of the niche signal that persists for a short period of time [2]. Our data suggest that this memory delays differentiation, allowing the cells to participate in stem cell replacement or to increase in number before committing to a cell fate choice. We are using quantitative fluorescence microscopy, clonal analysis, and high-throughput molecular and genetic methods to understand how cell fate is controlled during this labile period of differentiation.
The cell biology of cell fate decisions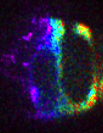
Although most studies of cellular differentiation focus on signaling pathways and transcription factors, many other aspects of cell biology are also important [1]. Our lab is interested in understanding how physical and chemical properties of cells such as pH [2], cytoskeletal dynamics [3], and cell shape [4] contribute to the regulation of cell fate decisions.
Most recently, we have discovered that an increase in intracellular pH occurs spontaneously during differentiation in the follicle stem cell lineage and is required for proper cell fate specification. Our collaborators found that increased intracellular pH is also required for mouse embryonic stem cell differentiation, indicating that changes in intracellular pH promote differentiation in diverse cell types. Little is known about how changes in pH influence cell fate decisions, but changes in pH are known to influence many other processes in the cell, including metabolism, cytoskeletal dynamics, and epigenetics. Thus changes in pH may help to coordinate multiple processes throughout the cell in response to a differentiation cue. We are building on our original observations and conducting forward genetic screens to investigate the role of pH in differentiation. Future studies will also address whether changes in pH are important in other stem cell lineages and whether other changes in the physical and chemical properties of cells also contribute to the regulation of cellular differentiation.